The SNaP System: Biomechanical and Animal Model Testing of a Novel Ultraportable Negative-Pressure Wound Therapy System
Kenton D. Fong, M.D., Dean Hu, M.S., Shaundra Eichstadt, B.S., Deepak M. Gupta, M.D., Moshe Pinto, M.B.A., Geoffrey C. Gurtner, M.D., Michael T. Longaker, M.D., M.B.A., H. Peter Lorenz, M.D., Stanford and Sunnyvale, Calif.
Background:
Negative-pressure wound therapy is traditionally achieved by attaching
an electrically powered pump to a sealed wound bed and applying
subatmospheric pressure by means of gauze or foam. The Smart Negative
Pressure (SNaP) System (Spiracur, Inc., Sunnyvale, Calif.) is a novel ultraportable
negative-pressure wound therapy system that does not require an electrically
powered pump.
Methods:
Negative pressure produced by the SNaP System, and a powered
pump, the wound vacuum-assisted closure advanced-therapy system (Kinetic
Concepts, Inc., San Antonio, Texas), were compared in vitro using bench-top
pressure sensor testing and microstrain and stress testing with pressure-sensitive
film and micro�computed tomographic scan analysis. In addition, to test in vivo
efficacy, 10 rats underwent miniaturized SNaP (mSNaP) device placement on
open wounds. Subject rats were randomized to a system activation group (approximately
�125 mmHg) or a control group (atmospheric pressure). Wound
measurements and histologic data were collected for analysis.
Results:
Bench measurement revealed nearly identical negative-pressure delivery
and mechanical strain deformation patterns between both systems. Wounds
treated with the mSNaP System healed faster, with decreased wound size by
postoperative day 7 (51 percent versus 12 percent reduction; p < 0.05) and had
more rapid complete reepithelialization (21 days versus 32 days; p < 0.05). The
mSNaP device also induced robust granulation tissue formation.
Conclusions:
The SNaP System and an existing electrically powered negativepressure
wound therapy system have similar biomechanical properties and
functional wound-healing benefits. The potential clinical efficacy of the SNaP
device for the treatment of wounds is supported. (Plast. Reconstr. Surg. 125:
1362, 2010.)
Disclosures:
K.D.F., D.H., and M.P. are current
employees of Spiracur, Inc., but were not employed by
Spiracur at the time of the animal experiments.
M.T.L. and H.P.L. are scientific advisors to Spiracur,
Inc., and have equity interests. The other authors
have no financial interests to disclose.
From the Division of Plastic and Reconstructive Surgery,
Department of Surgery, Stanford University School of Medicine,
Stanford University; the Department of Bioengineering,
Stanford University; and Spiracur, Inc.
Received for publication October 13, 2009; accepted November
20, 2009.
Copyright �2010 by the American Society of Plastic Surgeons
DOI: 10.1097/PRS.0b013e3181d62b25
Negative-pressure wound therapy for treatment of acute and chronic wounds has shown great efficacy, and numerous publications support its clinical use.1�6 In traditional negative-pressure wound therapy, a gauze or foam dressing directly contacts the wound bed, and an electrically powered pump is connected to a sealed enclosure over the wound. Mechanical stimulation has been theorized to be a key mechanism of action in negative-pressure wound therapy, leading to changes in biochemical signaling pathways, release of growth factors, and increased cellular proliferation.1,6�9 Saxena et al. described how negative pressure draws the wound surface into the open pores of the wound dressing foam, which creates regions of variable stress and strain.1 The wound surface in contact with the foamstruts is compressed, causing compressive stress and strain. Repeating patterns of high-strain gradients are created along the surface of the wound that appear as two-dimensional undulations across the wound surface.1 In addition, a fluidbased mechanism has been previously proposed as a contributing factor to the effectiveness of negative-pressure wound therapy. Negativepressure wound therapy removes excess interstitial fluid, leading to a decrease in interstitial pressure. Once the interstitial pressure drops below capillary pressure, capillaries are decompressed and are able to then reperfuse wound tissue.6 Thus, an effective negative-pressure wound therapy device must deliver mechanical stimulation and handle wound fluid dynamics within an appropriate negative-pressure range.
Recently, a novel ultraportable negative-pressure wound therapy system called the SNaP System was developed. The SNaP System consists of five basic elements: the vacuum/exudate cartridge, activation/ reset key, hydrocolloid dressing layer, extension tubing, and a gauze wound interface layer. Figure 1 shows photographs of the SNaP System (generation 1.0) used in this study. The commercially available version (generation 2.0) of the SNaP System has been further refined and simplified and can be seen in Figure, Supplemental Digital Content 1, http://links.lww.com/PRS/A155 (the yellow �75 mmHg model is shown). Compared with the earlier version of the device, there was an elimination of the button system, streamlining of the cartridge design, the addition of a red cartridge full/air leak indicator, and development of a customized hydrocolloid dressing layer. To create negative pressure without an electrically powered pump, a set of specialized constantforce springs causes forced air expansion within the system. A predetermined level of negative pressure is delivered in a constant fashion as exudate collects. With exudate inflow, the unique spring system moves the piston and expands the system volume to maintain the desired amount of negative pressure delivered to the wound. The disposable cartridge is produced with three different preset pressure levels (approximately �75, �100, and �125 mmHg), and is small enough to be worn on a patient�s leg, arm, or belt and hidden under clothing. Once placed on the patient, the SNaP System is left in place between dressing change visits and continues to deliver negative pressure unless the cartridge fills with exudates or there is an air leak. If the patient has a highly exudative wound that fills the capacity of the cartridge, the patient can change the cartridge at home. This type of negative- pressure wound therapy delivery system has several potential advantages over traditional pumps, including increased mobility, silent operation, and decreased cost.
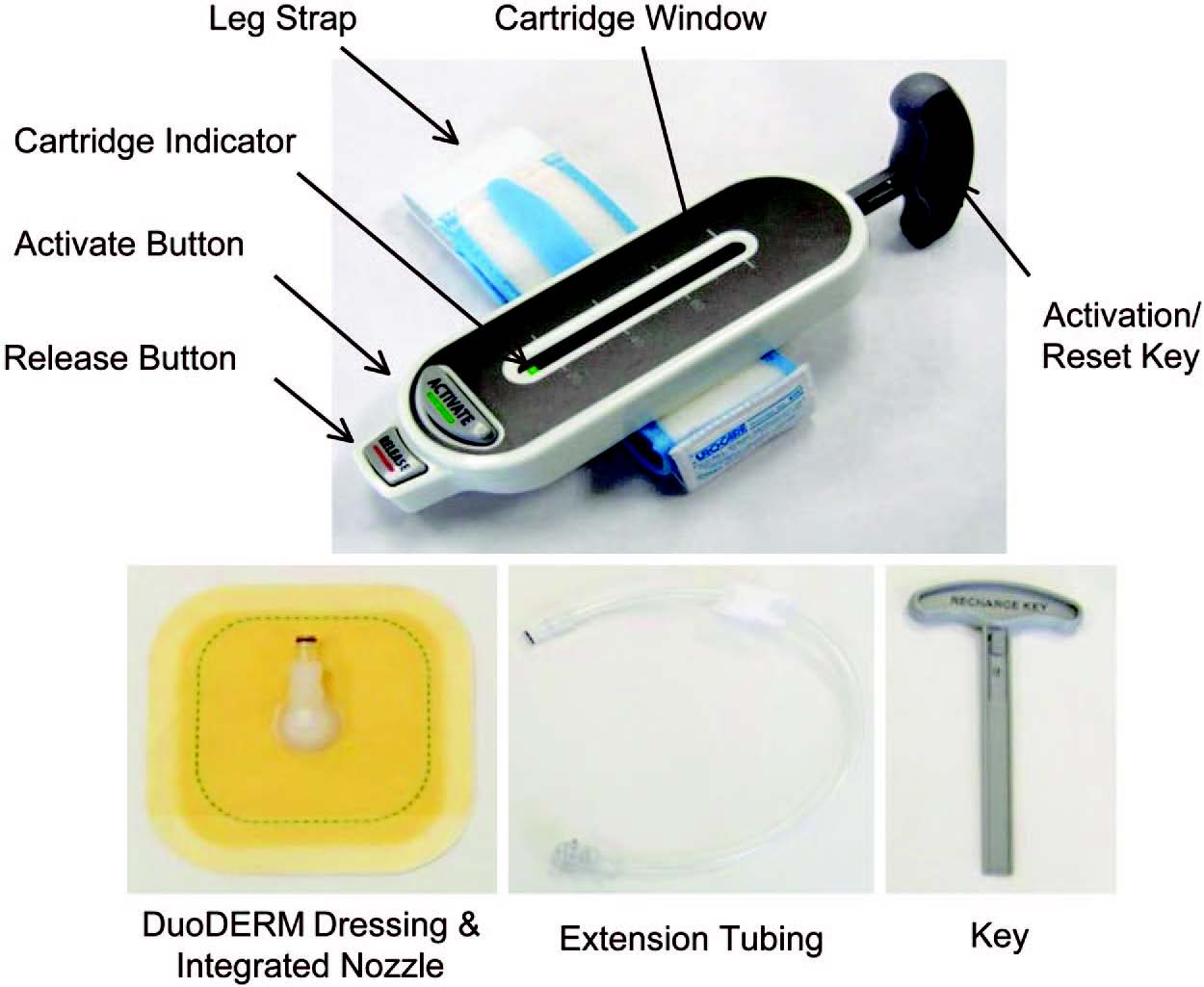
Fig. 1. The ultraportable SNaP Wound Care System (generation 1.0). When the activation button is depressed, negative pressure is delivered from the retraction of the sliding seal by constant-force springs to deliver a predetermined level of negative pressure.
This study compared the SNaP System to a benchmark negative-pressure wound therapy product, the wound vacuum-assisted closure advanced- therapy system device (Kinetic Concepts, Inc., San Antonio, Texas), an electrical diaphragm- pump system. The vacuum-assisted closure device creates negative pressure by removing mass from the system and uses foam instead of gauze as the wound interface layer. The vacuumassisted closure device uses continuous pumping to compensate for pressure loss from pump backstreaming and wound exudate inflow. This study examined the negative pressure delivered by both systems in a static state and with wound exudate present. The mechanical stress and strain patterns produced at the wound bed by each system was also compared. To evaluate the effect of negative-pressure wound therapy delivered by the SNaP System on wound healing in an in vivo model, a miniaturized version of the SNaP System (mSNaP System) was created and tested in a rat open wound model developed by Isago et al.3 Using a vacuum-assisted closure system in their model, Isogo et al. found significantly smaller wound areas in animals treated with �50, �75, and �125 mmHg of pressure compared with those treated with no pressure or �25 mmHg of pressure.3
This study used a foam product for both the vacuum-assisted closure and SNaP System experiments solely for purposes of comparison of operation of the two systems. Because both electrical diaphragm-pump and forced expansion mechanisms generate negative pressure at the wound bed, we hypothesized that the end effects of negative pressure (i.e., exudate evacuation, mechanical deformation, mechanical stimulation of the wound, and ultimately faster wound healing) should be the same.
Materials and methods
Biomechanical Testing
Pressure Measurement
A simulated wound-dressing enclosure was
constructed by placing a 6 x 6 x 3-cm piece of
GranuFoam (Kinetic Concepts) on a polycarbonate
plate. The foam was covered with a dressing
layer fitted with a pressure delivery port, connected
to either a vacuum-assisted closure device
or SNaP System set to deliver �125 mmHg of negative
pressure [see Figure, Supplemental Digital
Content 2, which demonstrates the biomechanical
pressure testing setup of the SNaP System without
(above) and with (below) exudate simulation,
http://links.lww.com/PRS/A156 (a, negative pressure
provided by either vacuum-assisted closure
(above) or the SNaP System (below) at 125 mmHg;
b, pressure delivery port; c, dressing; d, foam contact
layer; e, data-logging manometer; f, simulated
exudates infused at 5 cc/hour)]. The underside of
the plate was equipped with a separate port where
fluid could be introduced into the system; a datalogging
manometer was connected to monitor
pressure at the underside of the foam. The pressure
delivery system was activated and pressure
level was logged every 20 seconds (4000 over 24
hours = 4000/24/60/60 = 0.0463 per second =
1 every 20 seconds) for 24 hours.
The above procedure was repeated with the addition of simulated wound exudate, a mixture of 50 percent glycerol and 50 percent water (see Figure, Supplemental Digital Content2B, which shows a schematic of this set-up, http://links.lww.com/PRS/A156). The mixture was loaded into a 60-cc syringe pump (New Era Systems, Wantagh, N.Y.) connected to the underside port of the test plate and infused at 5 cc/hour. The vacuum-assisted closure device or SNaP System was connected and activated at �125 mmHg, and pressure measurements were recorded every 20 seconds for 8 hours. The pressure delivery systems were massed (in the case of the vacuum-assisted closure device, only the collection canister was massed) both before and after the testing period to measure the amount of exudate collected.
Mechanical Stimulation
Both contact stress and strain were evaluated
for the vacuum-assisted closure device and SNaP
System. To evaluate contact stress, a simulated
wound bed was created by laying a sheet of pliable
thermoplastic elastomer onto a polycarbonate test
surface. A piece of PressureX Micro pressure-sensitive
film of 2 x 2 cm (Sensor Products, Inc.,
Madison, N.J.) was placed on top of the thermoplastic
elastomer. GranuFoam (6 x 6 x 3 cm) was
placed on top and the wound was sealed and connected
to either a vacuum-assisted closure device
or SNaP System [see Figure, Supplemental Digital
Content 3, which shows the experimental setups
for evaluating wound contact stress and for micro�
computed tomographic wound surface strain imaging,
http://links.lww.com/PRS/A157 (a, negative
pressure provided by either vacuum-assisted closure
(above) or SNaP System device (below) at 125
mmHg; b, pressure delivery port; c, dressing; d,
foam contact layer; e, pressure-sensitive film; f, simulated
tissue; g, simulated wound enclosure (above,
left) inside micro�computed tomographic scanner)].
Negative pressure (either �125 mmHg or
�75 mmHg) was applied for 120 seconds and the
pressure-sensitive film was analyzed. Four different
setups were performed: vacuum-assisted closure
device at �75 mmHg, vacuum-assisted closure
device at �125 mmHg, SNaP System at �75 mmHg,
and SNaP System at �125 mmHg.
Contact strain produced by the vacuum-assisted closure device and SNaP System was evaluated using micro�computed microtomographic scanning, following a protocol similar to that developed by Saxena et al.1 A simulated wound-dressing enclosure was constructed inside a polyurethane block 5.5 cm in diameter, and a 3x3x1-cm sheet of thermoplastic elastomer was placed inside the block. On top of this sheet, a piece of 3 x 3 x 1-cm GranuFoam was placed. A dressing with connection tubing was then applied. The enclosure was then placed on the imaging bed of an Imtek MicroCAT II CT/MicroSPECT Scanner (Siemens Corp., New York, N.Y.) with connection tubing leading outside the scanner (see Figure, Supplemental Digital Content 3B, which shows a schematic of this setup, http://links.lww.com/PRS/A157). The tubing was connected to either a vacuum-assisted closure device or SNaP System set to produce either �125 mmHg or �75 mmHg of negative pressure, or to no pressure source. The imaging bed was positioned and a scan of the enclosure was acquired at a resolution of 36 x 36 x 41 μm. Five scans were obtained: no negative pressure, vacuum-assisted closure at �75 mmHg, vacuum-assisted closure at �125 mmHg, SNaP System at �75 mmHg, and SNaP System at �125 mmHg. Scans were reconstructed in real time using MicroCAT software and imported into AMIRA (Visage Imaging, Carlsbad, Calif.) for processing. From the threedimensional data set, slices parallel and perpendicular to the simulated wound bed were extracted. Simulated tissue surfaces were examined for evidence of deformation (strain) patterns under the different conditions.
Animal Studies
All animal experiments were performed under
the Stanford University Animal Procedures
and Care Committee�s approved protocol and
were compliant with the guidelines specified in
the National Institutes of Health�s Guide for the Care
and Use of Laboratory Animals. All animals were
housed in the Veterinary Service Center at Stanford
Medical Center with 12-hour light/dark cycles
and ad libitum water and rodent chow
throughout the study period.
Study Groups
Ten Sprague-Dawley rats weighing between 200
and 250 g (similar in size and age to those used by
Isago et al.3) were used in this study, divided randomly
into two groups. The first group had the
mSNaP System placed on their wounds with activation
of negative pressure at approximately �125
mmHg. The second group had the system placed on
their wounds without activation of negative pressure.
mSNaP System Negative-Pressure Wound
Therapy and Sealant System
The mSNaP System used for animal testing is
shown in Figure, Supplemental Digital Content 4,
http://links.lww.com/PRS/A158 (A, constant-force
spring; B, modified syringe body; C, activation
valve; D, syringe plunger seal; E, hydrocolloid skin
dressing; F, on activation, the constant force
springs pull on the plunger, maintaining a constant
level of reduced pressure in the system as
exudates collect). The system consists of a modified
7-cc Epilor syringe (part no. C3601; Qosina,
Edgewood, N.Y.) with an activation valve at one
end and a constant-force spring connected to a
plunger. The syringe chamber is charged by evacuating
air using a 20-ml syringe and closing the
activation valve to lock the plunger in the charged
position. GranuFoam was used as the contact layer
to the wounds. A specially modified hydrocolloid
dressing (DuoDERM; ConvaTec, Skillman, N.J.)
was used as a dressing. The hydrocolloid dressing
had a nozzle integrated into it using a washer
system and ultraviolet light�cured epoxy (Loctite
3311; Henkel, Düsseldorf, Germany). After activation,
a valve was attached to the nozzle dressing.
The valve was then opened to create air/fluid
communication, thus delivering negative-pressure
wound therapy to the wound. Bench testing of the
mSNaP System demonstrated it capable of delivering
�125 ± 10 mmHg of negative pressure.
Surgical Procedure
A surgical wound-healing model was performed
as described previously by Isago et al.3
Briefly, the animals were anesthetized and their
backs were shaved and depilated. Wounds (2.5 x
3.0 cm) were excised through skin and panniculus
carnosus using a scalpel and surgical scissors. The
wounds of each animal were photographed and
measured along the vertical and horizontal
lengths of the body axis. Wounds were dressed
with an interface layer of GranuFoam with a hydrocolloid
cover sheeting, followed by connection
of the mSNaP System. Mastisol ointment (Ferndale
Laboratories, Ferndale, Mich.) was applied to
intact skin of the animals to improve adhesion of
the dressing. The hydrocolloid dressing edges
were reinforced further using large Tegaderm
dressings (3M, St. Paul, Minn.). The syringe portion
of the system was fixed to the animals� bodies
by half-inch paper tape. Dressings were checked
every 24 hours for all animals, with emptying and
recharging of the system for those randomized
to the system activation group. Wound closure
was defined as complete reepithelialization
without drainage and was evaluated on a daily
basis after discontinuation of SNaP treatment by
a plastic surgeon.
Histologic Evaluation
All dressings were removed on postoperative
days 4 and 7. Punch biopsy specimens (6 mm)
of the central portion of the wound bed were
harvested and fixed in 4% paraformaldehyde.
Animals were not killed to obtain punch biopsy
specimens of wounds. Routine hematoxylin and
eosin staining was used to examine granulation
tissue formation.
Statistical Analysis
To assess group differences, a two-sample t test
(two-tailed) or modified t test for uneven variance
was applied. The values were considered to be
significant at a level of p < 0.05.
RESULTS
Mechanical Testing Data
Similar Pressure Delivered by the SNaP
System and the Vacuum-Assisted Closure Device
When No Exudate Is Present
When set at �125 mmHg and tested over a
period of 24 hours, the vacuum-assisted closure
device delivered an average pressure of �124.9 ±
1.3 mmHg. The SNaP System delivered �120.7 ±
1.18 mmHg. Both systemsmaintained a steady level
of negative pressure throughout the test period, with
very small degrees of variability, as demonstrated in
Figure 2. The pressures were measured over the
duration of the test (4000 time point measurements).
The stated standard deviations were calculated
from all data points taken during the respective
tests and reflecthowwell the device (vacuum-assisted
closure advanced-therapy system or SNaP Cartridge)
maintained a steady-state pressure.
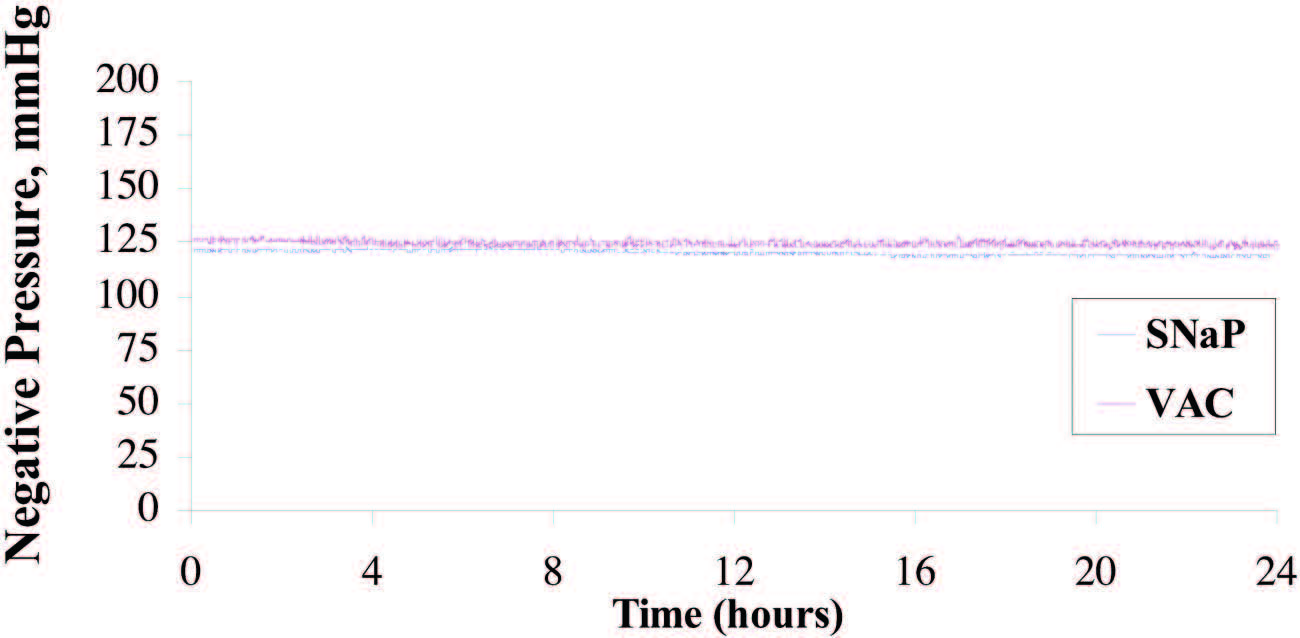
Fig. 2. Plot of pressure delivered under static state for SNaP System and vacuumassisted closure (VAC). The vacuum-assisted closure device delivered an average pressure of �124.9±1.3mmHg,whereas theSNaPSystem delivered �120.7±1.18mmHg. Both devices were able to maintain a steady level of negative pressure throughout the test period and demonstrated a very small degree of variability.
Similar Pressure Delivered by the SNaP
System and the Vacuum-Assisted Closure Device
When Exudate Is Present
Eight hours of pressure measurements were
recorded during the infusion of simulated exudate
at the rate of 5 cc/hour. The average pressure
delivered by the vacuum-assisted closure device
was 123.2 ± 0.8 mmHg, compared with 121.7 ±
3.1 mmHg delivered by the SNaP System. The
pressure signal of the SNaP System, although confined
to a tight band of negative pressure, exhibited
a sawtooth pattern characteristic of minute
movements of the constant-force spring mechanism
to correct for pressure loss during exudate
entry. A total of 40 cc of exudate was infused into
the simulated wound during the duration of the
test. The vacuum-assisted closure device collected
31 g, whereas the SNaP System collected
35 g of exudate. Figure 3 demonstrates that both
systems delivered and maintained a steady level
of negative pressure under conditions of fluid
introduction. The pressures were measured
over the duration of the test. The stated standard
deviations were calculated from all data
points taken during the respective tests and reflect
how well the device (vacuum-assisted closure
advanced-therapy system or SNaP Cartridge)
maintained a steady-state pressure.
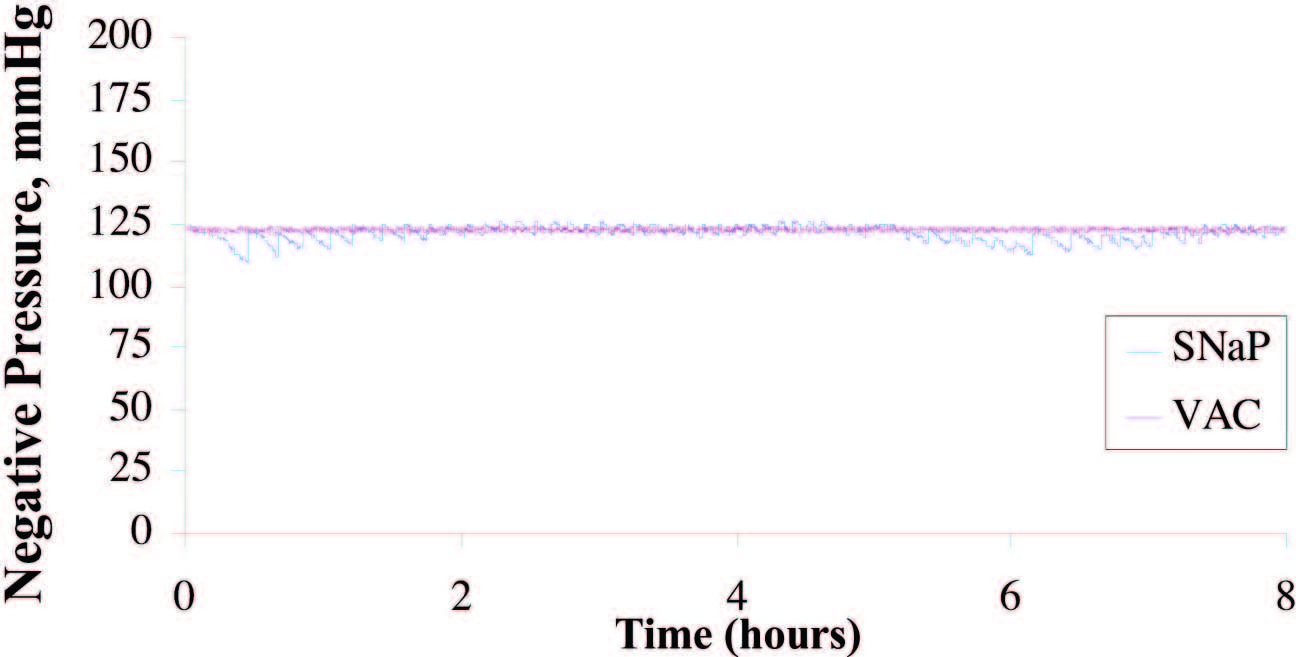
Fig. 3. Plot of pressure delivered during exudate test for SNaP System and the vacuum- assisted closure (VAC) device. Average pressure delivered by the vacuum-assisted closure device was �123.2 ± 0.8 mmHg; the SNaP System delivered �121.7 ± 3.1 mmHg. Both devices were able to deliver and maintain a steady level of negative pressure, even under conditions of fluid introduction.
Similar Contact Stress Patterns Delivered by
the SNaP System and the Vacuum-Assisted
Closure Device
The SNaP System and vacuum-assisted closure
device produced similar contact stress profiles for
equivalent pressure levels, as measured by micropressure-
sensitive film (Fig. 4). The patterns of
contact stress demonstrated that both systems conducted
negative pressure through the foam and
produced very similar wound surface stress gradients.
The pressure distribution image is qualitative,
showing relative areas of high stress and low
stress. Further quantitative analysis of the data was
precluded by the sensitivity limit of the pressuresensitive
film used.
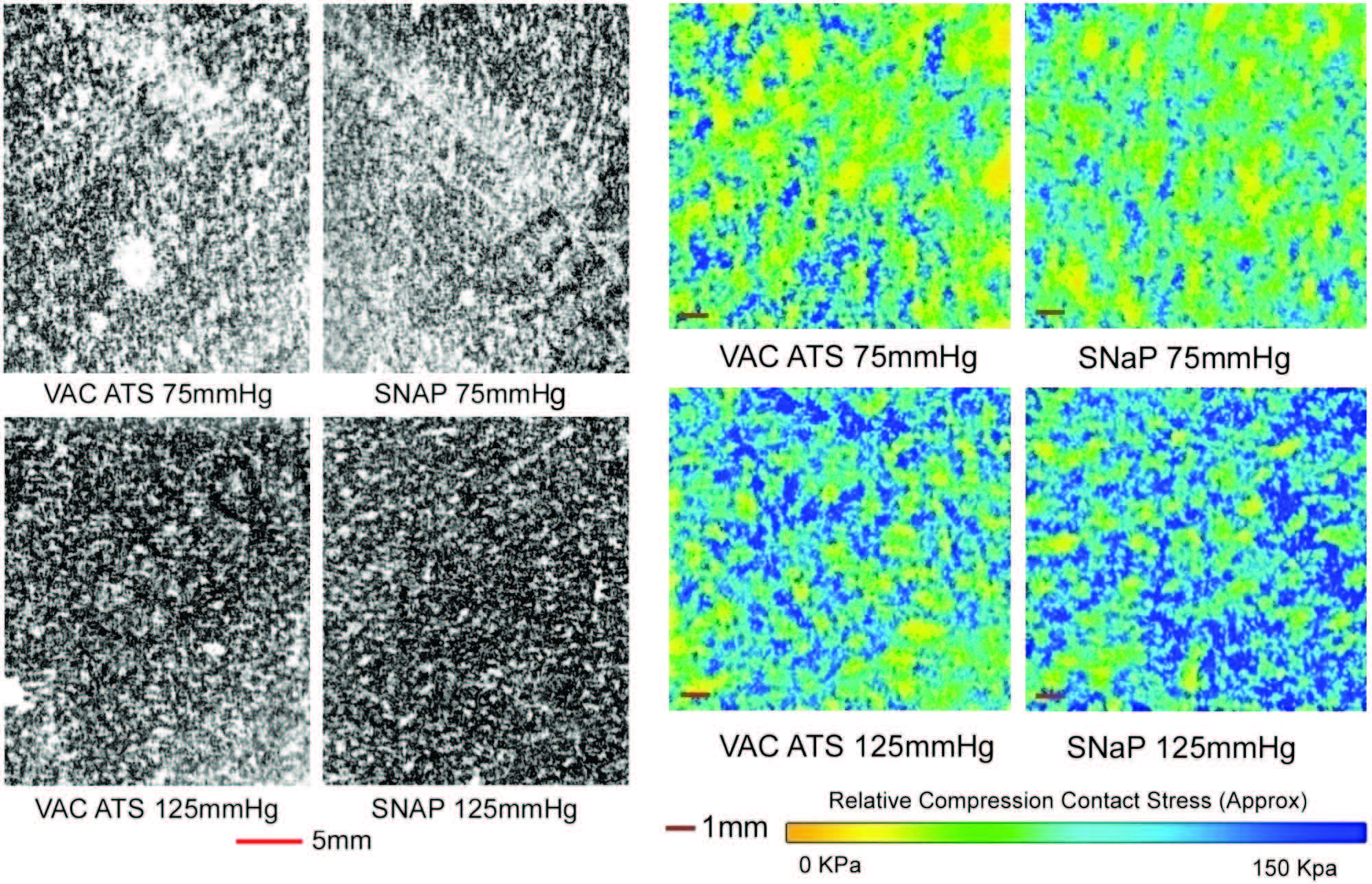
Fig. 4. Biomechanical testing, pressure measurement. (Left and second from left) Raw images of pressure-sensitive film recovered from under foam for vacuum-assisted closure (VAC) and the SNaP System at �75mmHgand �125 mmHg. Dark regions denote areas of high compressive stress in contact with film, and light regions denote no contact. Note that both the SNaP System and the vacuum-assisted closure device produce characteristic repeating patterns on length scales, consistent with foam pore size. (Right and second from right) Color-enhanced detail from pressure-sensitive films.
Similar Contact Strain Patterns Delivered by
the SNaP System and the Vacuum-Assisted
Closure Device
Notable features of the micro�computed tomographic
scans are shown in Figure 5. This figure
shows the foam microstructure to be clearly visible.
Without delivery of negative pressure, the simulated
wound bed/foam interface is smooth. With
delivery of negative pressure at �75mmHgor �125
mmHg by either the SNaP System or the vacuumassisted
closure device, the characteristic pattern
of deformation that occurs with negative pressure
occurs at the simulated wound bed/foam interface.
This pattern matches the predicted stress and
strain pattern of undulation described and demonstrated
by Saxena et al.1 No discernible difference
in the microdeformation patterns was observed
between the two pressures tested for either
the SNaP System or vacuum-assisted closure�
treated experiments. Furthermore, the patterns of
deformation appeared nearly identical for both
the SNaP System and vacuum-assisted closure at
the pressure levels tested. Because this was a qualitative
measure, no further quantitative analysis
was performed.
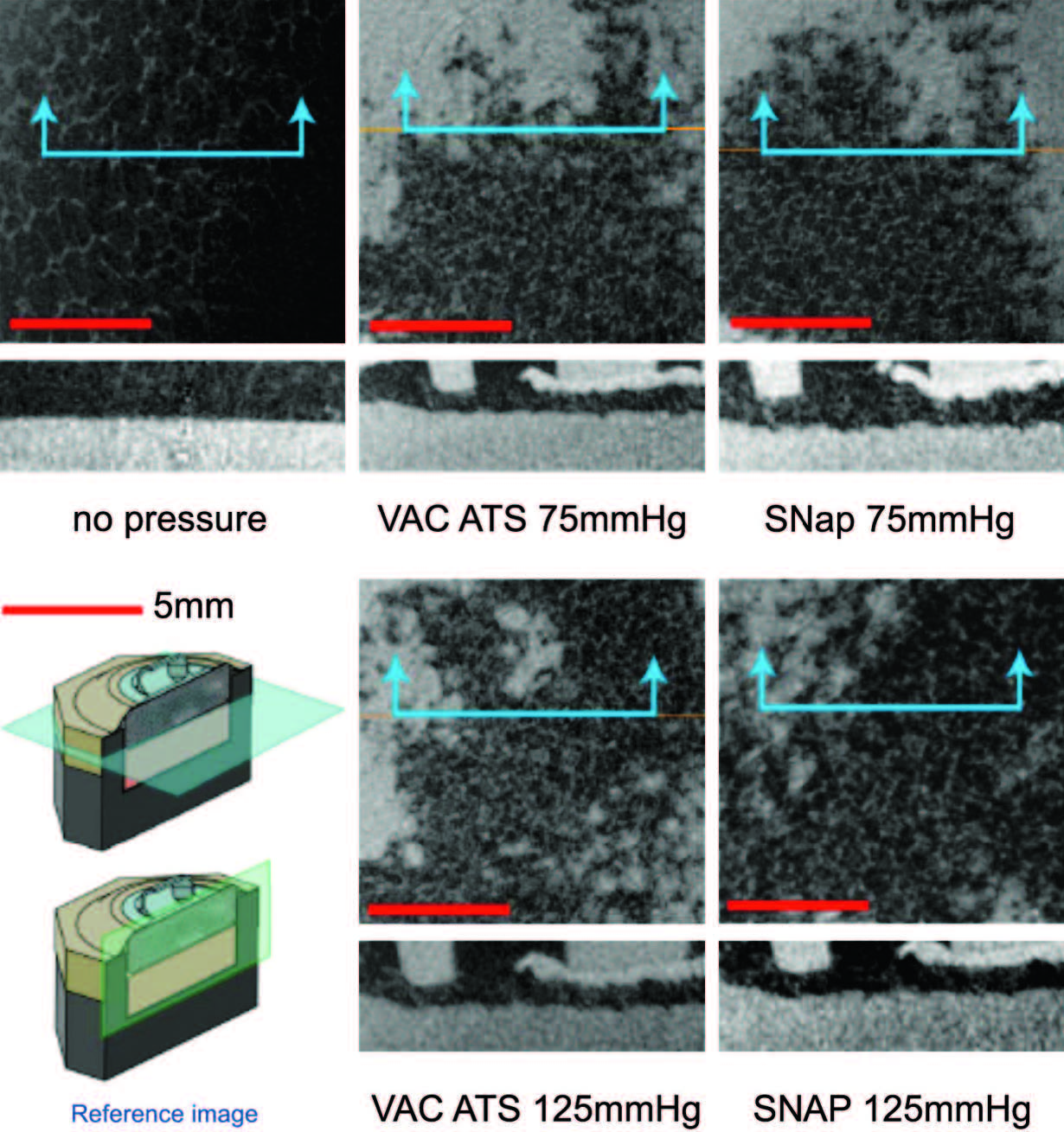
Fig. 5. Biomechanical testing, mechanical stimulation. Micro�computed tomographic scans parallel to the simulated tissue surface (top images, horizontal plane in reference image) and perpendicular (bottom images, vertical plane in reference image) for all tested pressure configurations. Note that both theSNaPSystemand the vacuum-assisted closure device produce similar characteristic repeating patterns at both pressures.
Animal Testing Data
Wounds Healed Faster with the Activated
mSNaP System
Animals treated with themSNaPSystem had a 51
percent reduction in wound size compared with a 12
percent reduction in wound size of control subjects
at 7 days (p < 0.05) (Fig. 6). Complete reepithelialization
also occurred faster than in control subjects
(21 days versus 32 days; p<0.05), as shown in Figure 7.
See Figure, Supplemental Digital Content 5, which
shows representative wounds at postoperative days 0
and 7, http://links.lww.com/PRS/A159 (note the increased
granulation tissue and smaller size of the
activated system�treated wounds compared with atmospheric
controls). Comparison of animals treated
with the mSNaP System to data from the study by
Isago et al. using the vacuum-assisted closure device
in the same animal model reveal very similar results.
ThemSNaPSystem�treated wounds demonstrated a
51 percent decrease in wound size, which is comparable
to the reported 40 percent decrease in wound
size observed for vacuum-assisted closure�treated
animals at 1 week.3 Control animals treated with
atmospheric pressure in this study and the study by
Isago et al. were 12 percent and 14 percent surface
area size reduction, respectively.3
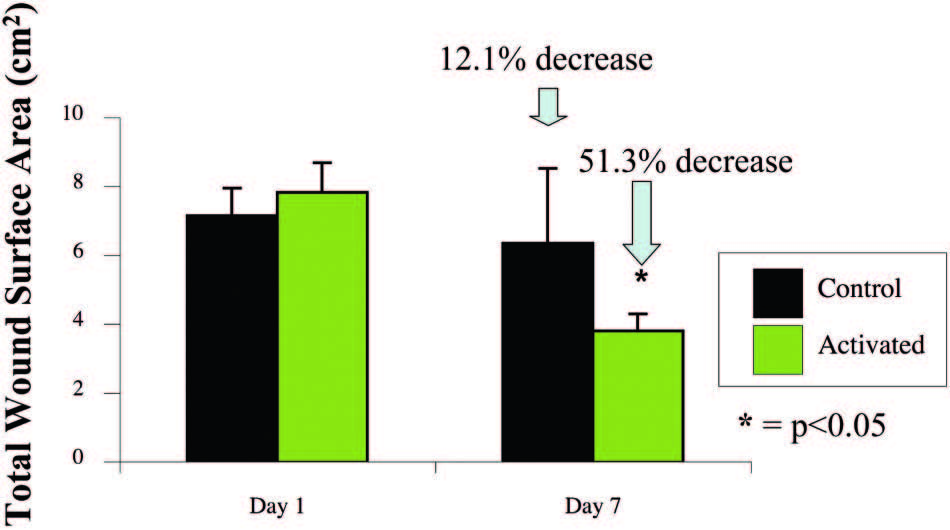
Fig. 6. The SNaP System decreases wound size. Negative-pressure wound therapy�treated animal wounds were significantly smaller than control wounds. Treated animals had 51 percent smaller wounds compared with 12 percent smaller wounds in control subjects on postoperative day 7 (p < 0.05).
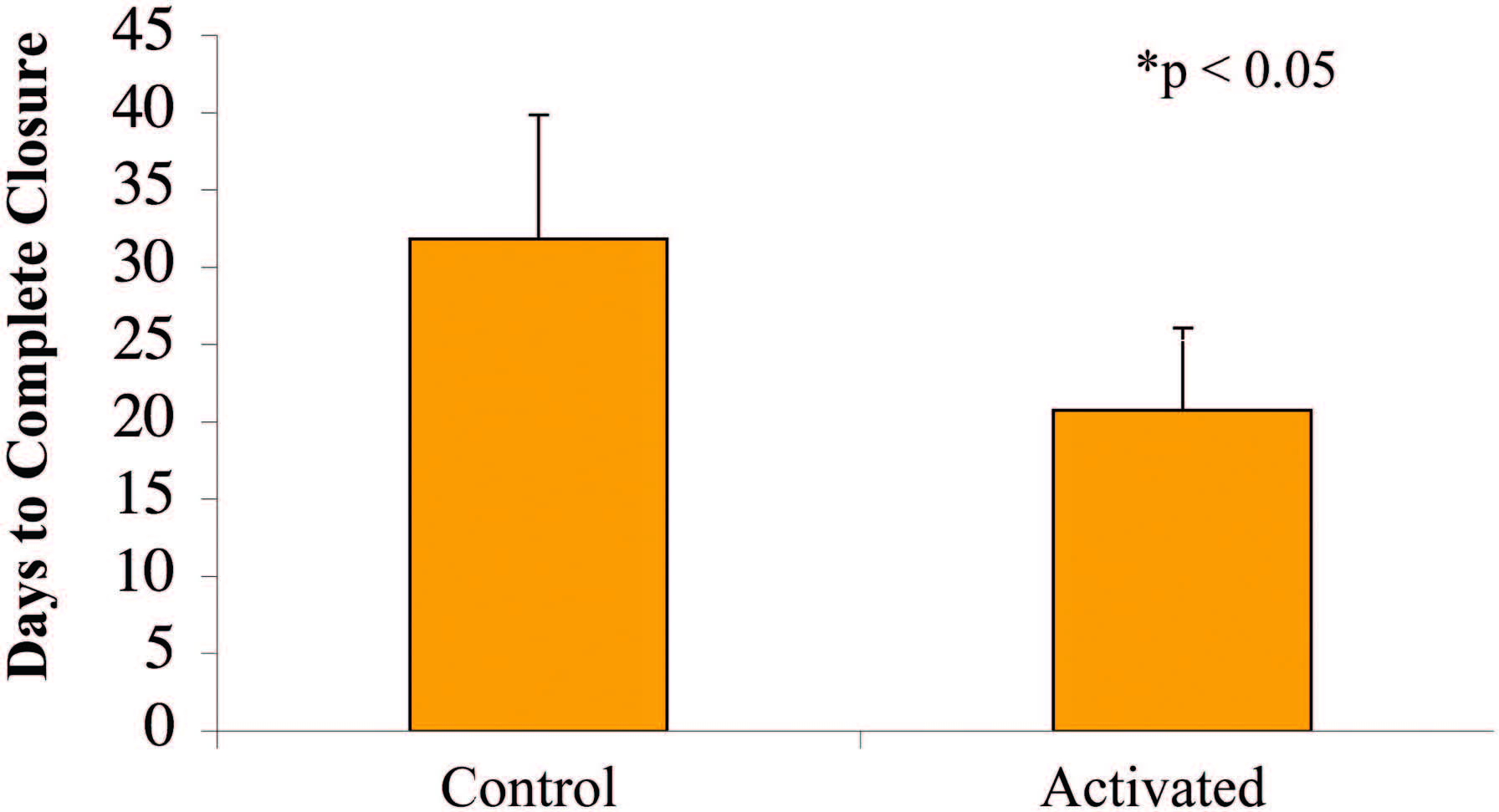
Fig. 7. Wounds treated with the SNaP System for 7 days healed faster (mean, 21 days) than control animal wounds (mean, 32 days) (*p < 0.05).
Wounds Had Greater Granulation Tissue
with the Activated mSNaP System
Similar to previous reports for the vacuumassisted
closure device,10 the mSNaP System promoted
granulation tissue formation compared
with controls, noted by gross examination of the
wounds (see Figure, Supplemental Digital Content
5, http://links.lww.com/PRS/A159) and by hematoxylin
and eosin staining on postoperative
days 4 and 7 (Figs. 8 and 9).
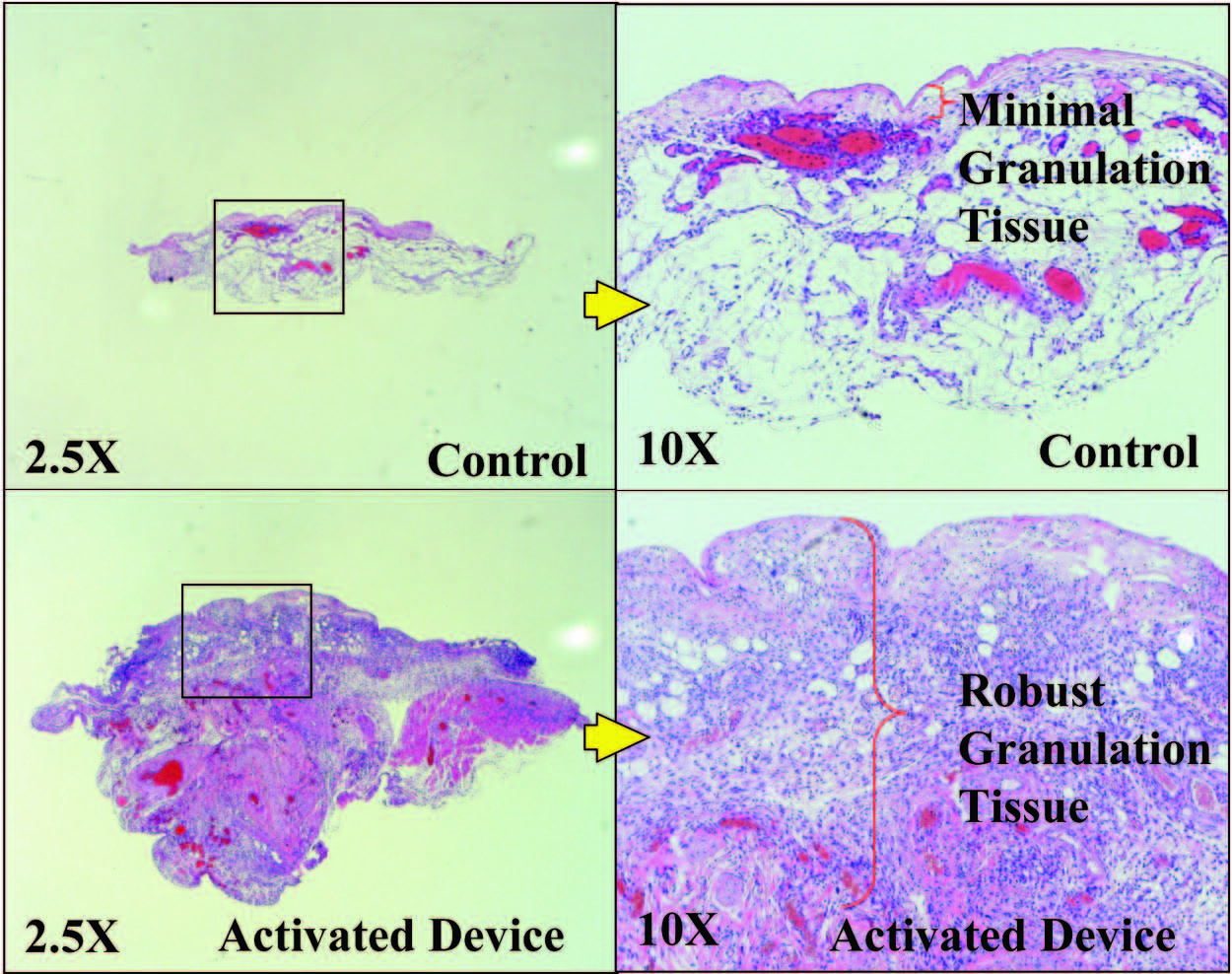
Fig. 8. Representative histologic sections demonstrating increased granulation tissue with SNaP treatment on postoperative day 4.
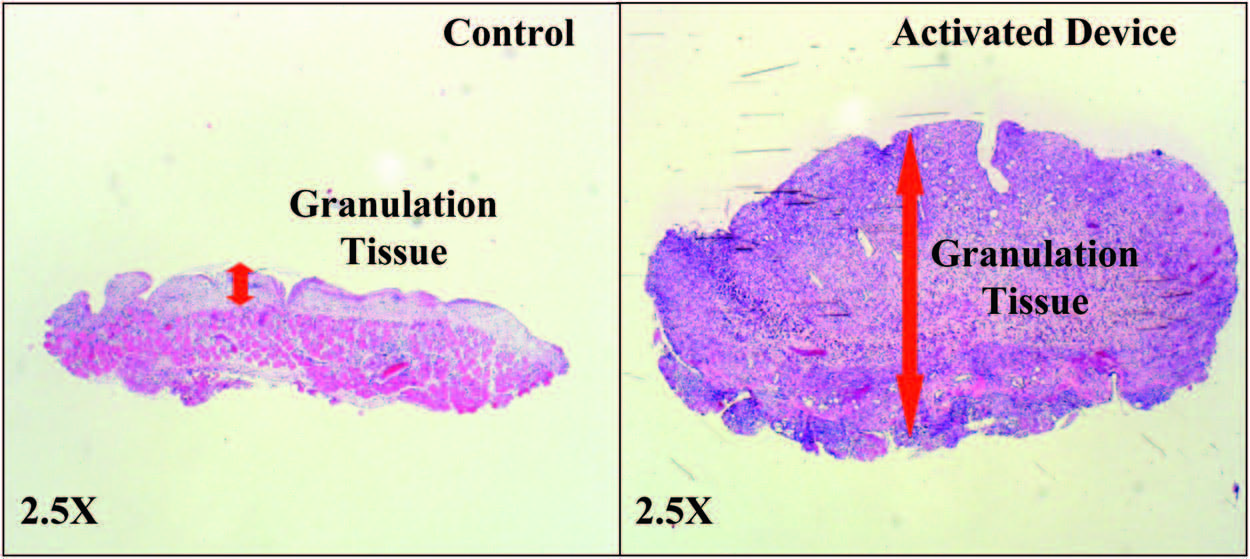
Fig. 9. Representative histologic sections demonstrating increased granulation tissue with SNaP treatment on postoperative day 7.
There were no fatalities, wound infections, or other significant complications from treatment in any of the animal studies. However, the DuoDERM seal did fail in two rat subjects (on postoperative days 3 and 6). The seal was reestablished with the addition of Tegaderm and paper tape to the area of dressing dehiscence.
DISCUSSION
In this study, a novel, ultraportable negativepressure
wound therapy system was evaluated. The
mechanical testing experiments demonstrated
that the SNaP System delivers steady negative pressure
to wound beds with and without exudate
present in a fashion similar to the vacuum-assisted
closure device. The mechanical stress and strain
patterns produced by the SNaP System were also
comparable to those created by the vacuum-assisted
closure device. Thus, from a pressure delivery
standpoint, the negative pressure delivered by
both systems was essentially identical. Based on
these findings alone, the SNaP System would be
expected to deliver the same functional benefits to
a wound as the vacuum-assisted closure device. To
test this hypothesis, the SNaP System was evaluated
in vivo using a rat open wound model. The SNaP
System delivered effective negative-pressure
wound therapy, evidenced by increased granulation
tissue formation and faster healing, consistent
with published results using vacuum-assisted
closure in the same animal model.3
Although human efficacy data are still needed for the SNaP System, and although foam is currently not recommended for use with the SNaP System, the biomechanical and in vivo data suggest that the SNaP System may have efficacy equal to that of vacuum-assisted closure for some wounds. This study was performed in a rodent model, and many differences exist between acute rodent wound healing and wound-healing problems found in humans. In addition, only a single pressure level, �125 mmHg, was tested in vivo. Other pressure levels may have worse, equal, or better wound repair outcomes. However, the potential benefits of a silent, disposable, less expensive, and less cumbersome negative-pressure wound therapy system may prove to be valuable to clinicians.
ACKNOWLEDGMENTS
This work was supported by Spiracur, the Oak Foundation,
and the Hagey Laboratory for Pediatric Regenerative
Medicine. Portions of the experiment (micro�computed
tomographic strain evaluation) were performed at the
Stanford Center for Innovation in In Vivo Imaging with
collaboration from Dr. Timothy Doyle (Stanford Center for
Innovation in In Vivo Imaging). Spiracur and SNaP are
trademarks of Spiracur, Inc. All rights reserved.
REFERENCES
- Saxena V, Hwang CW, Huang S, Eichbaum Q, Ingber D, Orgill DP. Vacuum-assisted closure: Microdeformations of wounds and cell proliferation. Plast Reconstr Surg. 2004;114: 1086�1096; discussion 1097�1098.
- Argenta L, Morykwas MJ. Vacuum-assisted closure: A new method for wound control and treatment. Clinical experience. Ann Plast Surg. 1997;38:563�577.
- Isago T, Nozaki M, Kikuchi Y, Honda T, Nakazawa H. Effects of different negative pressures on reduction of wounds in negative pressure dressings. J Dermatol. 2003;30:596�601.
- Armstrong DG, Lavery LA. Negative pressure wound therapy after partial diabetic foot amputation: A multicentre, randomised controlled trial. Lancet 2005;366:1704�1710.
- Vuerstaek JD, Vainas T, Wuite J, Nelemans P, Neumann MH, Veraart JC. State-of-the-art treatment of chronic leg ulcers: A randomized controlled trial comparing vacuum-assisted closure (V.A.C.) with modern wound dressings. J Vasc Surg. 2006;44:1029�1037; discussion 1038.
- Morykwas MJ, Simpson J, Punger K, Argenta A, Kremers L, Argenta J. Vacuum assisted closure: State of basic research and physiologic foundation. Plast Reconstr Surg. 2006;117 (7 Suppl):121S�126S.
- Wilkes R, Zhou Y, Cunningham K, Kieswetter K, Haridas B. 3D strain measurement in soft tissue: Demonstration of a novel inverse finite element model algorithm on MicroCT images of a tissue phantom exposed to negative pressure wound therapy. J Mech Behav Biomed Mater. 2009;2:272�287.
- Wilkes R, Zhou Y, Kieswetter K, Haridas B. Effects of dressing type on 3D tissue microdeformations during negative pressure wound therapy: A computational study. J Biomech Eng. 2009;131:031012.
- Orgill DP, Manders EK, Sumpio BE, et al. The mechanisms of action of vacuum assisted closure: More to learn. Surgery 2009;146:40�51.
- Morykwas MJ, Faler BJ, Pearce DJ, Argenta LC. Effects of varying levels of subatmospheric pressure on the rate of granulation tissue formation in experimental wounds in swine. Ann Plast Surg. 2001;47:547�551.